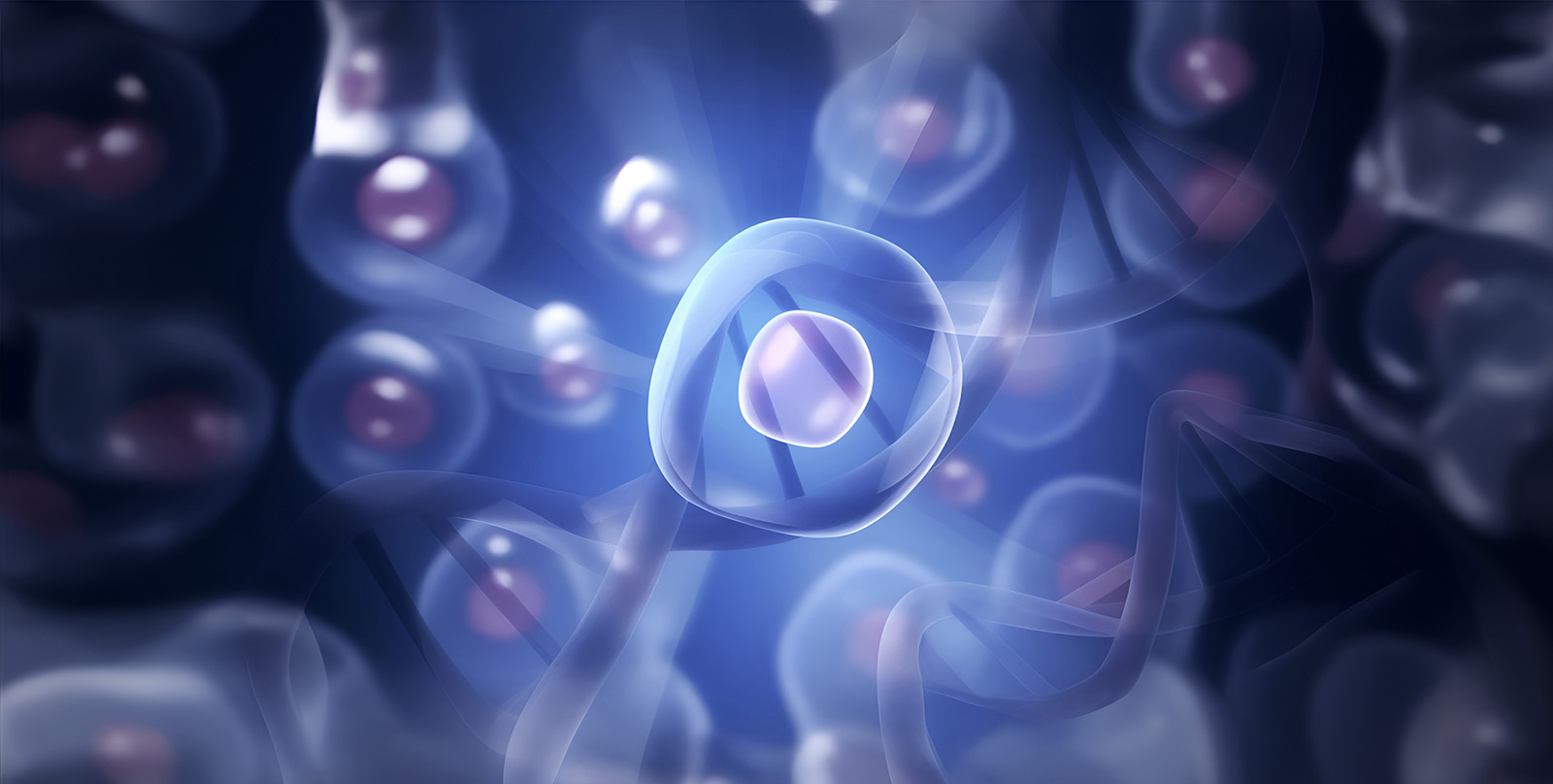
Total solutions for every patient
Photoreceptor cells are specialized nerve cells that act as light receptors, converting light into chemical signals and transmitting this visual information to secondary neurons, such as bipolar cells. These cells are located in the farthest layer of the retinal tissue within the eye, closest to the sclera (or the white part of the eye). They fall into two categories: rod cells, which facilitate vision in dim light, and cone cells, responsible for sharp vision and color perception. Cone cells are further divided into blue, green, and red types, based on their sensitivity to different light wavelengths, playing a crucial role in color vision. A distinguishing feature of photoreceptor cells is an intricate structure known as the outer segment, which efficiently captures light. This structure is densely packed with light-receptor molecules called opsins.
Age-Related Macular Degeneration (AMD) is one of the most common causes of blindness worldwide, particularly among the elderly. The global prevalence is 8.7% leading to an estimate that about 288 million people in western countries will be afflicted by 2040 (2). The early stages of AMD are characterized by features known as drusen and pigment loss from retinal pigment epithelium (RPE) cells. The progression of AMD is marked by the widespread degeneration of RPE cells and the formation of choroidal neovascularization (CNV). Advanced cases, directly linked to a decrease in quality of life (QOL) through central visual field impairment and visual impairment, are classified into two forms: atrophic and exudative (as shown below). In exudative forms, CNV leads to blood and lipid leakage beneath the retina, and fibrous scarring, which cause the degeneration of visual cells and visual impairment. Presently, the treatment consists of intravitreal injections of anti-vascular endothelial growth factor (anti-VEGF) agents for the exudative type, and complement inhibitory drugs for the atrophic type. These treatments aim to curb disease progression but require regular long-term intervention. However, neither treatment can restore visual function once the retina has already degenerated. Therefore, for the progressive cases of AMD, reconstructing the retina through cell transplantation remains the only means to preserve or restore visual function.
先進諸国における高齢者の主な失明原因の1つであるAMDの世界的な有病率は8.7%で、2040年までにグローバルで約2億8800万人が罹患すると予測されています。AMDの初期段階は、ドルーゼンおよびRPE細胞の色素脱失として認められ、進行するに従いRPEの広範囲な変性や脈絡膜血管新生(CNV)が形成されます。中心視野と視力障害というQOL低下に直結する視機能障害を特徴とする進行症例は、萎縮型と滲出型の2つの形態に分類されます。滲出型ではCNVが原因となり血液、脂質の網膜下漏出、および網膜下での線維性瘢痕の形成することで視細胞が変性し、視機能障害がおこります。現在、病状の進行抑制を目的とした対症療法として、滲出型に対して抗血管内皮成長因子(抗VEGF)剤、萎縮型に対して補体活性抑制薬の硝子体内注射が行われていますが、進行抑制のためには長期にわたる定期的な治療が必要となります。しかしながら、いずれの治療法においても既に変性を来した網膜の視機能回復は期待することはできません。そのため、AMDの進行症例に対する視覚機能を維持または回復するための根治治療には細胞移植による網膜再建が唯一の手段となります。
The Retinal Pigment Epithelium (RPE) is a single layer of cells that is formed by the hexagonal, brown-pigmented RPE cells arranged closely together like tiles or a mosaic. It is located at the outermost part of the retina. RPE cells directly interact with and protect the photoreceptor cells, which are critical components of the retina. These cells play an essential role in maintaining our vision. Specifically, they regenerate visual pigments to sustain the light sensitivity of photoreceptors through the visual cycle, secrete nutrient molecules to alleviate the stress induced by continuous exposure to light signals, and maintain the eye’s immunological isolation by forming the retinal blood barrier between the choroid and the retina. It is known that abnormalities in RPE cells (RPE deficiency) can cause a variety of retinal degenerative diseases, including age-related macular degeneration. Unfortunately, even with the advancement of medical technology today, there is no way to heal retina cells that have degenerated or disappeared.
The iPS cells used in our regenerative medicine can be broadly divided into autologous cells and allogeneic (heterologous) cells. Autologous cell therapy is a treatment method in which cells collected from the patient themselves are cultured and processed, then returned to the patient. While this method can avoid risks such as immune rejection reactions and infectious diseases caused by transplanted cells, making it safe, it requires cell culture and processing for each patient, resulting in a substantial amount of time and cost required for the treatment per patient. Moreover, due to individual differences in cellular characteristics, there may be variations in the quality of the final product. To address these challenges, researchers are developing cost-effective methods for producing autologous iPS cells, including automating the cell manufacturing process.
On the other hand, allogeneic (heterologous) cells are collected from a chosen healthy donor (another person) for transplantation, which may cause an immune rejection reaction. However, cells that meet certain quality standards can often be preserved for the long term, either as raw materials or as cultured and processed intermediates known as cell stock. This preservation may include freezing, among other methods. When compared to autologous cells, this approach can potentially reduce the time, cost, and quality variations associated with treatment, making allogeneic cells more suitable for practical use. Additionally, efforts are in progress to prepare iPS cells with specific alterations to the HLA, which is responsible for rejection reactions. These changes, made through genetic editing, aim to further enhance the feasibility and speed of practical applications.
網膜色素変性(RP)は、遺伝的背景により暗所での視機能を司る桿体視細胞が進行性に変性する疾患群であり、さらに進行すると中心視野、視力、色覚など司る錐体視細胞も2次的な変性をきたし、完全失明に至ることもある疾患です。現在では250もの原因遺伝子が報告されていますが、それらの遺伝子機能が解っていないものが多いです。その遺伝形式については常染色体優性、常染色体劣性、X連鎖性など、原因遺伝子により全ての形式が報告されていますが、家系内に他の発症者が確認できない孤発例もあります。原因遺伝子や生活環境によりますが、おおよそ20代〜40代で発症し、5年から10年に掛けて徐々に症状が進行します(幼少期から10代で発症される方もおられます)。
再生医療で用いるiPS細胞は、大きくは自家細胞と同種(他家)細胞に分けられます。自家細胞は、患者本人から採取した細胞を培養・加工し、本人に戻す治療法であり、免疫拒絶反応や移植細胞に起因する感染症のリスクを回避できるため、安全である反面、患者ごとに細胞の培養・加工が必要となるため、患者一人当たりの治療に必要な時間とコストが膨大となります。また、個人間の細胞性質の違いにより、最終製品の品質にばらつきが出る可能性があります。しかしながら、これらの課題に対しiPS細胞製造の自動化など自家iPS細胞を低コストで作成する技術開発がおこなわれています。
一方で、同種(他家)細胞は、選ばれた健康なドナー(他人)から採取された細胞を移植するため、免疫拒絶反応が起こる可能性がありますが、一定の品質基準に適合した細胞を原材料として、または培養・加工を施したものを中間体(細胞ストック)として長期保存(凍結保存等)することが多くの場合可能であるため、自家細胞と比較して治療にかかる時間とコスト、そして品質のばらつきを抑えることが可能となり、実用化に適しているといわれています。また、実用化をさらに加速させるために、拒絶反応の原因となるHLAの一部を遺伝子編集により破壊したiPS細胞の準備が進んでいます。
網膜色素上皮(retinal pigment epithelium: RPE)は、六角形で茶色い色素を持つRPE細胞が敷石状に配列することで形成された一層の細胞層であり、網膜の一番外側に存在します。RPE細胞は網膜の重要な部分である視細胞と直に接し、それを保護することで、私たちの視機能を維持するために重要な役目を担っています。具体的には、視色素を再生して視覚サイクルを介して光受容体の光感受性を維持し、栄養分子を分泌して光信号に常にさらされることによって引き起こされるストレスを軽減し、脈絡膜と網膜の間に網膜血管障壁を形成することで目の免疫学的隔離を維持します。そのため、RPE細胞の異常(RPE不全状態)が、加齢黄斑変性症等の実に様々な網膜変性疾患の原因になることが知られています。残念ながら、医療技術が発達した現在でも、変性・消失してしまった網膜細胞を治癒する方法はありません。
先進諸国における高齢者の主な失明原因の1つであるAMDの世界的な有病率は8.7%で、2040年までにグローバルで約2億8800万人が罹患すると予測されています(2)。AMDの初期段階は、ドルーゼンおよびRPE細胞の色素脱失として認められ、進行するに従いRPEの広範囲な変性や脈絡膜血管新生(CNV)が形成されます。中心視野と視力障害というQOL低下に直結する視機能障害を特徴とする進行症例は、萎縮型と滲出型の2つの形態に分類されます(下写真)。滲出型ではCNVが原因となり血液、脂質の網膜下漏出、および網膜下での線維性瘢痕の形成することで視細胞が変性し、視機能障害がおこります。現在、病状の進行抑制を目的とした対症療法として、滲出型に対して抗血管内皮成長因子(抗VEGF)剤、萎縮型に対して補体活性抑制薬の硝子体内注射が行われていますが、進行抑制のためには長期にわたる定期的な治療が必要となります。しかしながら、いずれの治療法においても既に変性を来した網膜の視機能回復は期待することはできません。そのため、AMDの進行症例に対する視覚機能を維持または回復するための根治治療には細胞移植による網膜再建が唯一の手段となります。
RPE dysfunction, which encompasses various retinal degenerative conditions like crystalline retinopathy, Best’s disease, Stargardt disease, retinitis pigmentosa (with related genetic defects like MERTK or RPE65), high myopia, multifocal retinal pigment epitheliopathy, and pigmentary striae, currently doesn’t have any definitive cure. Nevertheless, there’s a form of gene therapy, targeting RPE65 gene mutations associated with protein deficiency in retinitis pigmentosa. It was approved in the U.S in 2017, and in Japan in 2023. This treatment shows promise, but it’s important to note that it’s effective only if administered early in the disease progression. So, for patients with advanced stages of the disease, this therapy might not be applicable.
For patients with AMD, the current approach is routine medical management which involves the use of antioxidant supplements and anti-VEGF agents. Supplements including vitamins, lutein, and zeaxanthin are utilized to shield retinal cells from oxidative stress. Anti-VEGF agents, on the other hand, are used to treat wet AMD. They work by blocking VEGF’s interaction with its receptors, thereby preventing abnormal blood vessel growth. Despite these interventions, since they don’t address the underlying cause of the disease, stopping the treatment can often lead to high rates of disease recurrence.
There has been some progress in treating dry AMD, with the recent approval of an anti-complement drug. This drug is anticipated to slow down the progression of Geographic Atrophy (GA), a condition associated with dry AMD. However, it’s not capable of reversing already lost retinal cells. So, the current state of treatment for RPE dysfunction is such that there are only a handful of targeted gene therapies for specific types of RPE dysfunction. There’s still no cure for the common problem of RPE cell degeneration and loss that’s seen in both inherited and non-inherited forms of the disease. Some research groups, including ours, are investigating regenerative treatments, like cell transplantation, as potential new approaches to treating these conditions (see references 1 and 2 for details)
本国内で網羅的な遺伝子診断スクリーニングにより診断がつくのは4割から5割とされています。RPは国の指定難病の一つであり、その罹患者数は4000人から8000人に1人とされ、日本国内では約3万人、世界的には約300万人と言われています。
この病気では、多くの場合、最初に現れる症状は、暗いところでものが見えにくくなる夜盲です。その後、見える範囲(視野)が周辺部分から中心に向かい狭くなっていく視野狭窄が少しずつ進行していきます。さらに症状が進むと、視力の低下や、色の区別がつきにくくなる色覚異常が起きてくることが多いです。病気が進む速さは患者さんによって異なります。また、症状の組み合わせや順番にも個人差があり、最初に視力の低下や色覚異常で発見され、後から夜盲を自覚する患者さんもいます。
RPには現在確立した治療法はなく、対症的に遮光眼鏡の使用や、ビタミンAや循環改善薬の服用などが行われることがあります。遺伝子治療や人工網膜が実用化されつつありますが、今のところ、広く行うことができる治療法とはなっていません。私たちは、iPS細胞から作製した視細胞を含む網膜シートを、視細胞が変性した患者さんの網膜に移植することにより、視機能を回復させることを目指す再生医療を開発しています。また、原因遺伝子が網膜色素上皮(RPE)の遺伝子の場合はRPEを移植することで視機能を維持することができる治療も計画しています。
Initially, iPS cells were created from skin cells, but subsequent studies have shown that they can be generated from various types of cells. Nowadays, it’s more common to derive them from blood cells, making the process as simple as drawing blood. The genes used to induce iPS cells have also been modified, and the methods used to introduce these genes have evolved from retroviruses to plasmids and Sendai viruses. Today, iPS cells can even be created using mRNA. However, it’s important to note that iPS cells form a heterogeneous group, meaning they can greatly differ from each other. Even when generated using the same process, individual variations can lead to significant differences in gene expression levels. Moreover, the same cell line can behave differently depending on the cell culture techniques used. Even when following the same protocol, the cells can change differently during maintenance culture and passage, emphasizing the complexity of working with these cells.
During our pionnering work with iPS cells, we leveraged insights from the broader field of stem cell research to guide the transformation of these iPS cells into Retinal Pigment Epithelial (RPE) cells. While iPS cells are heterogenous and exhibit significant differences even within the same line, when they are differentiated into RPE cells, these differences become insignificant. Historically, there are no instances of these RPE cells turning cancerous, making them a safe choice for our work. We devised a robust method that ensures the creation of consistently safe RPE cells with identical functionalities from each patient’s unique iPS cells(1). This strategic approach made it possible for autologous transplants, effectively bypassing potential issues of immune rejection.
Subsequently, utilizing the iPS cells created by the Center for iPS Cell Research and Application (CiRA) at Kyoto University that matched the 6-locus HLA of a patient, we derived RPE cells and successfully transplanted them into the retina of an elderly patient with age-related macular degeneration. Notably, this procedure was carried out without the use of systemic immunosuppressive drugs[2]. The findings suggest that, when there is a precise HLA match, potential rejection reactions can be efficiently controlled through local steroid administration alone.
Exploiting the insights gained from these clinical trials, we are currently developing treatments that could allow us to transplant cells into any patient without requiring immunosuppressive drugs. This involves creating RPE cells from iPS cells that have undergone genetic alterations to partially disable specific HLA genes, thereby creating a universal cell line applicable to all patients.
Reference:
(1)Characterization of human induced pluripotent stem cell-derived retinal pigment epithelium cell sheets aiming for clinical application. Kamao H, Mandai M, Okamoto S, Sakai N, Suga A, Sugita S, Kiryu J, Takahashi M. Stem Cell Reports. 2014 Jan 23;2(2):205-18.
(2)HLA-Matched Allogeneic iPS Cells-Derived RPE Transplantation for Macular Degeneration. Sugita S, Mandai M, Hirami Y, Takagi S, Maeda T, Fujihara M, Matsuzaki M, Yamamoto M, Iseki K, Hayashi N, Hono A, Fujino S, Koide N, Sakai N, Shibata Y, Terada M, Nishida M, Dohi H, Nomura M, Amano N, Sakaguchi H, Hara C, Maruyama K, Daimon T, Igeta M, Oda T, Shirono U, Tozaki M, Totani K, Sugiyama S, Nishida K, Kurimoto Y, Takahashi M. .J Clin Med. 2020 Jul 13;9(7):2217.
最初は皮膚細胞から作られたiPS細胞ですが、その後は様々な細胞から作れることが報告され、現在では血球細胞から作られることが多くなり採血するだけで作れるようになっています。導入遺伝子の改変も行われ、導入方法もレトルウイルスから始まり、プラスミド、センダイウイルスと報告され、現在ではmRNAでもiPS細胞が作れます。しかし、iPS細胞はヘテロな集団であり、同じ製法であっても個体差も大きく遺伝子発現レベルでは性質が大きく異なります。たとえ同じ細胞株でも細胞技術が異なれば同じプロトコールでも維持培養や継代の際に異なった状態に変化してしまいます。
私たちが世界初のiPS細胞の応用例を行った際に、これらの幹細胞研究の経験から、網膜色素上皮(RPE)細胞という転移性腫瘍の報告が歴史上全くない細胞に純化することによって、患者ごとに違うiPS細胞であっても常に同じ機能を持つ安全な細胞を作れるように頑健な(robustな)製法を採用しました(1)。この方法によって自家移植を可能とし、免疫拒絶を回避することができたのです。その後、京都大学iPS細胞研究所(CiRA)が作製したHLA6座ホモのiPS細胞を用いてHLA6座適合の他家iPS-RPE移植を全身的免疫抑制剤の投与なしに高齢の加齢黄斑変性患者の網膜下に移植し、すなわちHLA非適合の影響がなければ局所のステロイドのみで拒絶反応を完全にコントロールして生着させることに成功しています(2)。この経験をもとに、現在ではHLAの部分的にKO(KnockOut)したiPS細胞を用いてRPEを作成することにより、すべての患者さんに1つの細胞株で免疫抑制剤なしに移植できる治療を準備しています。
参考文献:
(1)Characterization of human induced pluripotent stem cell-derived retinal pigment epithelium cell sheets aiming for clinical application. Kamao H, Mandai M, Okamoto S, Sakai N, Suga A, Sugita S, Kiryu J, Takahashi M. Stem Cell Reports. 2014 Jan 23;2(2):205-18.
(2)HLA-Matched Allogeneic iPS Cells-Derived RPE Transplantation for Macular Degeneration. Sugita S, Mandai M, Hirami Y, Takagi S, Maeda T, Fujihara M, Matsuzaki M, Yamamoto M, Iseki K, Hayashi N, Hono A, Fujino S, Koide N, Sakai N, Shibata Y, Terada M, Nishida M, Dohi H, Nomura M, Amano N, Sakaguchi H, Hara C, Maruyama K, Daimon T, Igeta M, Oda T, Shirono U, Tozaki M, Totani K, Sugiyama S, Nishida K, Kurimoto Y, Takahashi M. .J Clin Med. 2020 Jul 13;9(7):2217.
Retinal organoids are three-dimensional constructs that replicate the structure of in vivo retinas. They can be produced in vitro from embryonic stem (ES) cells or induced pluripotent stem (iPS) cells, facilitated by specific soluble factors that induce differentiation. Interestingly, these organoids mimic the natural progression of retinal development, establishing a complex three-layered structure comprising the outer nuclear layer, inner nuclear layer, and ganglion cell layer. During this process, all cell types, including photoreceptor cells, differentiate from retinal progenitor cells.
Several culture and differentiation methods exist, each utilizing different soluble factors. A popular strategy involves guiding differentiation from ES/iPS cells to the retina via the neural ectoderm. Our research employs a method utilizing Bone Morphogenetic Protein 4 (BMP4), part of the Transforming Growth Factor-beta (TGFβ) family. We use the Serum-free Floating culture of Embryoid Body-like aggregates with quick reaggregation (SFEBq) technique, where ES/iPS cell aggregates are suspended, and BMP4 is applied to induce differentiation towards retinal cells. This approach has enabled the production of high-quality retinal organoids, and it was instrumental in the world’s first transplantation of an iPS cell-derived retinal sheet into a patient with retinitis pigmentosa in 2020 at Kobe Eye Center Hospital. Our ongoing efforts are centered around using this technique to develop larger retinal organoids for transplantation.
Retinal organoids differentiated from iPS cells of patients with retinal degenerative diseases offer potential tools for pathology analysis and for developing treatments to halt disease progression. Our aim is to leverage retinal organoids as transplant tissues, targeting the restoration of lost vision and visual fields.
At present, our transplantation technique involves the use of retinal sheets that are derived from induced pluripotent stem (iPS) cell-generated retinal organoids. During transplantation, these sheets are primarily composed of retinal and photoreceptor precursor cells. Post-transplantation, these cells mature into photoreceptors and differentiate into secondary neurons such as bipolar and horizontal cells, as well as Müller glial cells. Our method of transplanting retinal sheets, which maintains the polarity of the immature tissue, has been found to offer consistent long-term integration and stability of the transplanted photoreceptor cells, a feature that distinguishes it from transplantation methods using purified photoreceptor cells in suspension.
However, there is a potential challenge that photoreceptor cells and secondary neurons differentiated within the transplanted retina may establish synaptic connections, thus inhibiting the formation of synapses between transplanted photoreceptor cells and the host’s secondary neurons. In response to this, we’re actively improving our transplantation strategies to maximize therapeutic outcomes. One of these enhancements involves the development of next-generation transplantation retinae, where gene-editing technologies are employed to regulate gene expression within the retina. By modifying the differentiation and maturation trajectory of the transplanted retina, our goal is to optimize the efficiency of synaptic connections between the host and transplanted retina.
Our transplantation process primarily utilizes retinal and photoreceptor precursor cells, which undergo post-transplantation maturation. This process establishes a light signal transmission pathway. Notably, electron microscopy reveals the formation of specialized light detection compartments, known as rod outer segments, after this maturation. Simultaneously, the cells express essential functional molecules intrinsic to photoreceptor cells, such as opsin and transducin. Our primate-based transplant trials further demonstrate the robust integration and enduring stability of transplanted photoreceptor cells, confirmed up to two years post-transplantation.
For the restoration of visual function, it is essential that transplanted photoreceptor cells are not only responsive to light stimulation, but also capable of transmitting light information to downstream secondary neurons. This involves the formation of ribbon synapses, a specialized structure that facilitates the transmission of graded light signals from photoreceptor cells to second-order neurons, such as bipolar cells. This process is critical for conveying light information. Beyond the evidence from immunohistochemistry, we have utilized electrophysiological techniques to demonstrate the light signal transmission capability of transplanted photoreceptors. By transplanting sheets derived from human retinal organoids into rats with immunodeficient retinal degeneration, we recorded the light response of the host retina using a multi-electrode array (MEA) system. These findings indicate that transplanted retinas are able to respond to light and transmit that information to host ganglion cells.
We have further assessed the restoration of vision by conducting behavioral experiments. In one experiment, retinal organoids derived from mouse iPS cells were transplanted into mice with end-stage photoreceptor degeneration. The transplant recipients exhibited a response to light in shuttle avoidance tests, reflecting a successful integration of the transplanted photoreceptor cells and their physiological response to light. In a separate study, human retinal organoid-derived sheet transplants were applied to monkeys with artificially induced photoreceptor degeneration. The results from visually-guided saccades (VGS) tests suggested noticeable improvements in the visual field, further affirming the potential of our transplant approach in restoring light-induced behavior.
One significant factor to consider in iPS cell transplantation is the recipient’s immune response. Our first clinical trial in 2014 employed autologous iPS cells, i.e., those derived from the patient’s own body, to minimize the risk of rejection. This method leverages the Human leukocyte antigen (HLA), a cell surface molecule instrumental in distinguishing self from non-self. The immune system eliminates cells bearing non-self HLA, ensuring the body’s protection. Therefore, in an autologous transplant, there’s no need for immunosuppressants to manage HLA-mismatch-induced rejection responses. However, this approach has its drawbacks; it requires extensive preparation time, labor, and substantial cost due to the need to establish iPS cells for each patient.
To overcome these challenges, the Center for iPS Cell Research and Application (CiRA) at Kyoto University launched the iPS Cell Stock Project for Regenerative Medicine. The project provides iPS cells from donors homozygous for the six major HLA loci, thereby reducing the likelihood of rejection in patients who share at least one pair of each of the six major HLA loci with the donor.
Our 2017 clinical trial used these HLA-matched RPE cells for transplantation. We demonstrated that such cells could be safely administered without necessitating systemic immunosuppressant use (1).
Despite the advantages of HLA-matched cells, this method is still limited to patients whose HLA type aligns with the six major HLA homozygous iPS cells provided by CiRA. As a solution, we established iPS cells using gene editing to remove part of the six major HLA loci and differentiated these into RPE cells (2). The eye exhibits stronger immunosuppressive properties than other organs, which further lowers the risk of rejection. In vivo and in vitro validations revealed no rejection responses to these modified RPE cells. By retaining some HLA, we maintain natural immunity against tumors and resistance to viruses, combining both safety and efficacy in suppressing the rejection response for intraocular transplantation.
Reference:
(1)Sugita S, Mandai M et al. J Clin Med 2020
(2)Ishida et al., in preparation
In the 2014 trial, our approach consisted of the formation of a sheet from iPS derived RPE cells(1,2). The advantage of this method was the precision it offered in graft placement, as the sheet was already formed before transplantation. However, this process requires a large incision to the retina during the procedure to introduce the large sheet.
Fast forward to 2017, we embarked on a different path. In the subsequent trial, the RPE cells were administered as a cell suspension. This format was simpler and more manageable in terms of cell preparation, storage, and required only a small surgical incision, thus causing less disturbance to the retina. However, this method came with its own challenges, such as ensuring the exact quantity of cells were delivered to the transplantation site.
To overcome the disadvantages of both cell sheet and cell suspension methods, while leveraging their benefits, we have developed the RPE strip [2]. By culturing RPE cells, differentiated from iPS cells, in narrow grooves for two days, we can form a strip-like aggregate, approximately 200μm in diameter and about 2cm in length. Once this strip is placed on a culture dish, we observe RPE cells starting to grow from it the next day. As the cultivation continues, these cells expand into a sheet while expressing mature RPE markers, such as BEST1 and ZO-1. Interestingly, it’s also possible to preserve the strip in its aggregated form.
In November 2022, we initiated clinical trials using the RPE strip, demonstrating its potential to be transplanted to targeted areas underneath the retina with minimal invasion, thereby complementing the shortcomings of both cell sheet and cell suspension methods. Furthermore, a few weeks post-transplantation, it was observed that the strip expands into a monolayer sheet, effectively integrating into the retina. Currently, we are considering the logistics, including transportation methods, to provide this strip formulation in a ready-to-use form for domestic and international use.
Reference:
(1)Mandai M, Watanabe A et al. N Engl J Med 2017
(2)Takagi S, Mandai M et al. Opthalmol Retina 2019
(3)Sugita S, Mandai M et al. J Clin Med 2020
(4)Nishida M., Tanaka Y., et al. Sci Rep 2021
当社の前身である理化学研究所 網膜再生医療研究開発プロジェクト(以下、網膜再生PJ)では、2014年、滲出型加齢黄斑変性の患者を対象に、患者自身のiPS細胞から分化誘導したRPE細胞をシート状に培養して網膜下へ移植するiPS細胞初の臨床研究を実施しました(1,2)。その後2017年には、京都大学iPS細胞研究所(CiRA)で作成された(他人の)HLA6座ホモのiPS細胞から分化させたRPE細胞を、細胞懸濁液の状態でHLA6座とも適合した患者さんに網膜下移植する臨床研究を行いました(3)。
RPE細胞は網膜下でシート状の組織となり機能しますが、移植の際にはなるべく眼球及び網膜の切開が小さい方が手術は安全です。最初からシートである方が移植片を移植部位に狙い通り投入することができる一方で、RPE細胞シートの作製に手間と時間を要し、また移植時の網膜の侵襲が大きくなる短所があります。それに対して、細胞懸濁液で投与して網膜下でシートを作らせる方法は、作製や保存が比較的容易であり、手術切開も小さな穴だけで良い一方、移植部位へ必要な細胞数を確実に投与することが困難となります。
細胞シートと細胞懸濁液の短所を補い、両者の長所を活かすべく開発されたのが、RPE凝集紐です(4)。iPS細胞から分化誘導したRPE細胞を、細い溝で2日間培養すると、直径約200μm、長さ約2cmの凝集塊ができます。その紐状の凝集塊を培養ディッシュにのせると、翌日以降、凝集紐からRPE細胞が生え出す様子が観察され、継続培養することでBEST1やZO-1といった成熟RPEマーカーを発現しながら細胞がシート状に広がる様子が認められました。また、凝集紐の状態で保存することも可能です。
2022年11月、RPE凝集紐の形で臨床研究が開始され、凝集紐が最小限の侵襲で、網膜下の狙った部位へ投与することができ、細胞シートと細胞懸濁液それぞれの短所を補完することが示されました。また、移植後数週間で単層のシート状に広がり、生着するのが認められました。現在はこの紐製剤を、Ready-to-useの形で国内外に提供するために、輸送方法を含め検討をおこなっています。
参考文献:
(1) Mandai M, Watanabe A et al. N Engl J Med 2017
(2) Takagi S, Mandai M et al. Opthalmol Retina 2019
(3) Sugita S, Mandai M et al. J Clin Med 2020
(4) Nishida M., Tanaka Y., et al. Sci Rep 2021
In 2014, our forerunner, the RIKEN Laboratory for Retinal Regeneration, led a landmark clinical application of induced pluripotent stem (iPS) cells. We carried out the world’s first subretinal transplantation of Retinal Pigment Epithelium (RPE) cells derived from iPS cells for treating neovascular age-related macular degeneration (1,2). This achievement marked the first application of human iPS cells since their discovery in 2006. To ensure safety and efficacy in this pioneering therapy, rigorous quality control tests, including whole-genome and exome analyses, were conducted. We managed to overcome numerous regulatory challenges, thus making a significant stride in the field.
The treatment involved procedures for neovascular removal and transplantation of iPS cell-derived RPE sheets. Throughout the process, no adverse events related to the surgical technique were observed. A year-long follow-up revealed no indications of abnormal proliferation or rejection of the transplanted cells. Furthermore, the grafted retinal structure was well maintained (1). Impressively, even seven years post-surgery, the transplanted RPE sheet, derived from iPS cells, remained intact without signs of tumorigenesis or abnormal cell proliferation.
The patient, a 78-year-old at the time of surgery, had previously received 13 intravitreal injections of anti-VEGF agents to suppress neovascularization due to exudative age-related macular degeneration. Remarkably, for over seven years post-surgery, the patient required no further intravitreal injections and maintained preoperative visual acuity.
Reference:
(1)Mandai M, Watanabe A et al. N Engl J Med 2017
(2)Takagi S, Mandai M et al. Opthalmol Retina 2019
Our company is propelling the techniques developed in the realm of basic research, undertaking the task of automating cell manufacturing through the use of robotics and AI. We are striving to devise processes that consistently generate high-quality cells suitable for clinical use. We’ve already achieved approval from the Committee for Designated Regenerative Medicine and the Health Sciences Council for clinical trials at Kobe Eye Center Hospital, where Maholo assists in part of the iPS-RPE production process.This innovative incorporation of robotics in clinical cell production has led to a more stable manufacturing process. By optimizing cell culturing techniques without altering human culture and protocols, we’ve been able to seamlessly transition this approach into clinical cell production.
Additionally, our cell culture processing facility, known as the Facility for iPS derived retinal stem cell therapy (FiRst, approximately 357 m²), received a “Special Cell Processing Product Manufacturing License” from the Kinki Bureau of Health and Welfare of the Ministry of Health, Labour and Welfare on October 20, 2022. FiRst is designed with two independent cell processing rooms, allowing for ongoing manufacturing in one room while updating equipment in the other, thereby ensuring smooth automation implementation and methodological enhancements. It is set to carry out cell manufacturing for future clinical trials.
人工多能性幹細胞(iPS細胞)とは、成人の細胞のエピゲノムを初期化(リプログラミング)して胚性幹細胞(ES細胞)のような状態にした幹細胞の一種です。このプロセスにより、細胞は体内のほぼすべての種類の細胞に分化する能力を持つようになります。(図1)
iPS細胞は、2006年に京都大学の山中伸弥教授らによって初めて作られました。山中教授の研究チームは、まず、細胞の多能性(あらゆる種類の細胞になる能力)を維持する鍵になると思われる24の遺伝子を選び出し、成人の皮膚細胞に挿入しました。一つずつ遺伝子を減らす消去法で、これらの成体細胞を胚の状態に再プログラムするのに必要な遺伝子は4つだけであることを発見しました(1)。(それらはOct3/4、Sox2、c-Myc、Klf4であり、現在では山中因子として知られています。)この発見は、体内のあらゆる細胞を胚から得られる多能性幹細胞ES細胞様の細胞に変える可能性があることを意味し、タイムマシンの様な研究と呼ばれる革命的な発見でした。iPS細胞は患者自身の細胞から作ることができるため、移植による拒絶反応のリスクを軽減した個別化治療、創薬、病気のモデル化などに利用できる可能性があり、爆発的に研究が広がりました。その功績が認められ、細胞の初期化(リプログラミング)の概念に関する先駆的な研究を行ったジョン・ガードン博士と共に山中教授は「2012年・ノーベル生理学・医学賞」を受賞し、その後も数々の賞を受賞しました。
参考文献:
図1:
Retinitis Pigmentosa (RP) is a set of genetic disorders leading to the gradual degeneration of rod cells, the photoreceptors responsible for vision in dim light. As the condition worsens, it can start to affect cone cells, which manage central vision, color perception, and sharpness of vision, possibly culminating in complete blindness. Currently, there are more than 250 identified genes associated with RP, although the specific function of many of these genes is not yet fully understood.
The pattern of inheritance can vary greatly depending on the causative gene, with all forms being reported: autosomal dominant, autosomal recessive, and X-linked. However, there are also isolated cases where no other instances of the condition can be traced within the patient’s family.
RPEは私たちの視覚を維持するために様々な重要な役割を担っていることから、RPEに発現する遺伝子異常や加齢に伴う酸化ストレス及び老廃物の異常蓄積等加齢に伴う病的変化が原因となり、RPE不全を来すことで遺伝性疾患群である網膜色素変性症や、これまでのRPE細胞移植臨床研究の対象疾患であったAMDの主な原因となることが知られています。事実、RPE不全が主な原因である網膜変性疾患が複数存在することから、これらの網膜変性疾患をRPE不全症という一つの疾患群としています。これらの疾患には、網膜色素変性症の類縁疾患であるクリスタリン網膜症や網膜色素変性症のうちRPE関連遺伝子異常を伴うもの等(RPE65、RDH5、MERTK等の遺伝子に異常を伴うもの)希少な遺伝性網膜変性疾患や、指定難病に登録されているものから高齢者における一般的な失明原因であるAMDも含まれます。AMDの患者数は日本国内で約70万人、世界では約3億人と言われています。一方で、他のRPE不全症に含まれる疾患の患者数はわかっていません。また、RPE不全症の症状は、視力が低下したり、暗いところでものが見えにくかったり、または色がわかりにくくなることで発見されますが、病気が発見される年齢や、症状の種類と進む速さは、その病気の種、類や患者さんによって個人差があります。RPE不全症の代表例としては加齢黄斑変性が挙げられます。
参考:
当社はこれまでの基礎研究分野における技術開発をさらに発展させ、細胞製造のロボット・AI化に取り組んでいます。臨床に用いる細胞を対象として、高品質な細胞を安定して製造するプロセスの開発に挑戦しています。すでに神戸アイセンター病院の細胞培養加工施設においてiPS-RPE製造の一部を「まほろ」が担う臨床試験について再生医療等特定認定委員会及び厚生科学審議会(再生医療等評価部会)の承認を得ており、ロボットを臨床用の細胞製造に投入することで安定した細胞製造ができるようにしています。人の培養とプロトコールを変えることなく細胞培養技術の最適化を図ることができたためにスムーズに臨床用細胞製造に応用できました。
また、当社の細胞培養加工施設 Facility for iPS derived retinal stem cell therapy(略称: FiRst 約357 m² )は、2022年10月20日付にて厚生労働省近畿厚生局より、再生医療等の安全性の確保等に関する法律(再生医療等安全性確保法)第35条第1項に基づく「特定細胞加工物製造許可証」(施設番号: FA5220002)を取得しています。FiRstでは、独立して空調管理を行える細胞調製室を2部屋備え、1つの部屋で製造を継続しながら他方の部屋で機器設備の改良/更新が可能。自動化装置の導入および製造法の改良がスムーズに行えるように設計されており、今後の臨床試験用の細胞製造を行います。
視細胞は光受容体として受け取った光を化学的シグナルに変換し、双極細胞などの二次ニューロンに光情報を伝える神経細胞で、視細胞は眼球内の網膜組織の最も外層(強膜側)に存在しており、薄明視を司る桿体視細胞と、視力や色覚を司る錐体視細胞の2種類のサブタイプに分類されます。錐体視細胞はさらに光の波長に対する感受性の違いから青、緑、赤錐体の3種類に分類され色覚をつかさどります。視細胞は外節と呼ばれる光を効率よく受け取るための特徴的な構造を有し、外節内には光受容分子であるオプシンが密に分布しています。
網膜色素上皮(retinal pigment epithelium: RPE)は、六角形で茶色い色素を持つRPE細胞が敷石状に配列することで形成された一層の細胞層であり、網膜の一番外側に存在します。RPE細胞は網膜の重要な部分である視細胞と直に接し、それを保護することで、私たちの視機能を維持するために重要な役目を担っています。具体的には、視色素を再生して視覚サイクルを介して光受容体の光感受性を維持し、栄養分子を分泌して光信号に常にさらされることによって引き起こされるストレスを軽減し、脈絡膜と網膜の間に網膜血管障壁を形成することで目の免疫学的隔離を維持します。そのため、RPE細胞の異常(RPE不全状態)が、加齢黄斑変性症等の実に様々な網膜変性疾患の原因になることが知られています。残念ながら、医療技術が発達した現在でも、変性・消失してしまった網膜細胞を治癒する方法はありません。
私たちはiPS細胞から分化誘導した網膜オルガノイドから切り出した組織片(網膜シート)を移植する方法を採用しています。網膜シートは移植時には主に網膜前駆細胞と視細胞前駆細胞から構成されており、移植後に成熟し、視細胞が形成されます。またその過程で双極細胞や水平細胞などの二次ニューロンや、ミューラーグリア細胞なども分化します。このような未分化な組織を極性を維持して移植する網膜シート移植では、視細胞を精製し懸濁液で移植する方法に比べ、長期に渡って安定的に移植視細胞が生着することが分かっています。
一方で移植網膜内で分化した視細胞と二次ニューロンがシナプス結合し、移植視細胞とホスト側の二次ニューロンとのシナプス結合を阻害するリスクが存在します。そこで現行の移植網膜を改良し、移植による治療効果を最大化するための検証を行っています。その1つとして、遺伝子編集技術を用いて網膜に存在する遺伝子の発現を制御した次世代型の移植網膜を作製しています。移植網膜の分化・成熟過程を改変することで、ホスト網膜とのシナプス結合効率の改善を目指します。
移植に用いる網膜オルガノイドは主に網膜前駆細胞と視細胞前駆細胞から構成されており、これらの細胞は移植後に成熟します。その結果、視細胞に外節構造が発達するとともにオプシンやトランスデューシンなどの機能分子も発現し、光報情伝達経路が発達します。また光情報を双極細胞を含む二次ニューロンに伝達するためのシナプスも形成されます。我々がヒト網膜オルガノイドから調整した網膜シートをモデル動物に移植したところ、移植視細胞は十分に成熟し、生体内の視細胞と同等の外節構造が発達することを電子顕微鏡を用いた観察で明らかにしました。光情報伝達に必要な機能分子が発現していることと、移植視細胞がホスト側双極細胞とシナプス結合していることを示唆する結果も免疫組織化学的手法により示しています。またサルを用いた移植試験から、移植後2年以上経過しても移植視細胞の生着状態が良好であることを確認しています。
移植視細胞がホスト網膜と機能的に繋がっていることを示すために、多電極電極アレイシステム(Multi-electrode-array, MEA)を用い電気生理学的に検証しました。具体的には視細胞が変性した末期の免疫不全網膜変性ラットにヒト網膜オルガノイドから調整した網膜シートを移植し生着させた後、ホスト網膜を移植網膜とともに取り出し、ホスト側神経節細胞の光に対する応答をMEAで記録しました。その結果、移植網膜が存在する部位でMEAの応答が検出されました。このことは移植視細胞が光に対して応答すること、さらにその信号がホスト網膜に伝達されていることを示唆しています。
私たちは移植により視機能の改善が見られるかを行動実験でも検証しています。マウスiPS細胞由来網膜オルガノイドを末期視細胞変性モデルマウスに移植後行った行動実験(shuttle aviodance test)では、網膜移植を行ったマウスが光に反応できたことから、光に対する移植視細胞の生理学的応答が行動に反映されることが示唆されました。また、人工的に視細胞変性を起こしたサルの網膜下にヒト網膜オルガノイドから調整した網膜シートを移植したケースでは、visually-guided saccades(VGS)テストにより視野の改善を示唆する結果が得られました。
網膜オルガノイドとは生体内の網膜”様”の構造を持つ立体組織で、ES細胞(胚性幹細胞)やiPS細胞(多能性幹細胞)からin vitroで液性因子を用いて分化誘導することで作り出すことができます。発生過程はin vivoの網膜発生と同様に進行します。また組織形成は細胞間の相互作用のもと自律的に進行し、外顆粒層、内顆粒層、神経節細胞層の3層構造が形成されます。この過程において、視細胞を含む全ての細胞種も網膜前駆細胞から分化すします。
接着培養や浮遊培養などの培養方法や、誘導方法に用いる液性因子に多少の違いがありますが、ES/iPS細胞から神経外胚葉を経由し網膜に誘導する分化方法が一般的です。我々の開発ではTGFβファミリーに属するBMP4による網膜分化誘導方法を用いている。Serum-free Floating culture of Embryoid Body-like aggregates with quick reaggregation(SFEBq)法がその代表的手法で、ES/iPS細胞の凝集塊を浮遊培養し、BMP4を用いて網膜への分化誘導刺激を加えます。揺り戻し法を組み合わせることで非常に高品質な網膜オルガノイドを作製することが可能になります。実際、神戸アイセンター病院で2020年に行われた、網膜色素変性患者への世界初のiPS細胞由来網膜シート移植にはこの手法を用いて作製された網膜オルガノイドが用いられました。我々はこの誘導方法をベースに開発された、より大型の網膜オルガノイドの作製技術を用いて移植用網膜を作製しています。
網膜変性疾患の患者由来iPS細胞から網膜オルガノイドを分化した場合は、その病態の解析に用いたり、疾患の進行を抑制するのに有効な治療薬の開発などに用いることができます。我々は網膜オルガノイドを移植組織として用いることで、失った視野や視力の回復を目指しています。
細胞膜表面分子であるヒト白血球抗原(Human Leukocyte Antigen; HLA)は多型性に富み、生体の免疫システムにおいて、自己と他者の区別に使われます。免疫システムは、自己の遺伝子でコードされてないHLAを膜表面に提示する細胞を非自己と認識して排除することで体を守る一方、移植医療においては、他者由来の移植片を異物とみなして排除してしまいます。これが、臓器(細胞)移植における拒絶反応です。
拒絶反応を回避するため、京都大学iPS細胞研究所(CiRA)では、再生医療用iPS細胞ストックプロジェクト[1]を立ち上げ、HLA主要6座(HLA-A, -B, -C, -DP, -DQ, -DR)がホモ接合対であるドナーから作製したiPS細胞を広く提供しました。これにより、HLA主要6座のすべてにおいて、遺伝子対の少なくとも片方が移植細胞と一致する患者は拒絶反応が起きにくくなります。我々の研究チームでも、2017年以降、CiRAが提供するHLA主要6座ホモ接合のiPS細胞とHLA型が一致する患者に対して、他家iPS細胞由来のRPE細胞移植を実施し、全身的に免疫抑制剤を投与しなくても拒絶応答を安全に管理できることを示しました[2]。
しかし、この方法は、CiRAが提供するHLA主要6座ホモ接合のiPS細胞と、HLA型が合う一部の患者にしか適用できませんでした。そこで我々は、独自にHLA主要6座の一部の発現を遺伝子編集により除去したiPS細胞を樹立し、RPE細胞に分化させました。眼は他の臓器に比べて免疫抑制性が強く、MHC主要6座のうち一部だけを除去したサルiPS細胞由来のRPE細胞を移植した in vivo 試験及び in vitro の検証で、拒絶反応は認められませんでした [3]。逆に、HLAの一部が残ることによって、腫瘍に対する自然免疫の発動が保持され、ウイルスに対する抵抗性が確保されます。少なくとも眼内移植においては、拒絶応答抑制と安全性の両面から有効と考えられます。
参考文献:
[1] Center for iPS Cell Research and Application (CiRA) iPS Cell Stock Project
https://www.cira.kyoto-u.ac.jp/e/research/stock.html
[2] Sugita S, Mandai M et al. J Clin Med 2020
[3] Ishida et al., in preparation
2014年、当社の前身の理研 網膜再生PJは、世界に先駆けて滲出型加齢黄斑変性の患者を対象に、患者自身のiPS細胞から作製したRPE細胞の、網膜下への移植を実施しました[1,2]。これは、2006年にiPS細胞が発明[3]されてから、世界で初めて実現したヒトiPS細胞の臨床応用で前例のない移植治療であったため、全ゲノムおよびエクソーム解析を含む厳しい品質管理検査がおこなわれ、多くの規制を乗り越えて、大きな第一歩が踏み出されました。
本臨床研究では、科学的に最も良い治療でありiPS細胞の特性を生かした自家iPS細胞由来RPE移植の安全性を確認することを目的としていました。新生血管抜去とiPS細胞由来RPEシートの移植が実施されましたが、手術手技に伴う有害事象はみられませんでした。その後1年間の経過観察において、移植片の異常増殖や拒絶を示す所見は認められず、 移植片上の網膜構造も良好に維持されていました[1]。また術後7年の時点でも、iPS細胞由来RPEシートは、移植された場所にとどまり、腫瘍化など異常な細胞増殖もみられていません。患者は、手術当時78歳で、滲出型加齢黄斑変性にともなう網膜下の血管新生を抑制するために、手術前に抗VEGF製剤の眼内注射を計13回受けていましたが、手術後は7年以上にわたって治療薬の眼内注射を受けることなく、手術前の視力を維持しています[4]。
自己のiPS細胞から作製された移植片をもちいる治療は、究極のテイラーメイド医療と言えます。移植細胞のHLAが患者自身の遺伝子でコードされているので、HLA不適合による拒絶応答を抑制するための免疫抑制剤を使用する必要がありません。特に高齢者に対する免疫抑制剤の使用は、患者に体力的な負担を強いることになり、その点において自己のiPS細胞を使用する大きな意義があります。その一方で、患者ごとにiPS細胞を樹立し、目的細胞を作製するプロセスは、大変な準備期間と労力、そして莫大なコストを要します。それらのバランスを考慮しながら、自己のiPS細胞をもちいた治療を必要とする患者に提供する仕組みが今後必要にとなります。
参考文献:
[1] Mandai M, Watanabe A et al. N Engl J Med 2017
[2] Takagi S, Mandai M et al. Opthalmol Retina 2019
[3] Tahakashi K and Yamanaka S. Cell 2006
[4]2023年3月24日 日本再生医療学会 神戸市立神戸アイセンター病院 栗本康夫氏による発表(本文執筆は こちらを参照)
私たちの再生医療で用いるiPS細胞は、大きくは自家細胞と同種(他家)細胞に分けられます。自家細胞は、患者本人から採取した細胞を培養・加工し、本人に戻す治療法であり、免疫拒絶反応や移植細胞に起因する感染症のリスクを回避できるため、安全である反面、患者ごとに細胞の培養・加工が必要となるため、患者一人当たりの治療に必要な時間とコストが膨大となります。また、個人間の細胞性質の違いにより、最終製品の品質にばらつきが出る可能性があります。しかしながら、これらの課題に対しiPS細胞製造の自動化など自家iPS細胞を低コストで作成する技術開発がおこなわれています。
一方で、同種(他家)細胞は、選ばれた健康なドナー(他人)から採取された細胞を移植するため、免疫拒絶反応が起こる可能性がありますが、一定の品質基準に適合した細胞を原材料として、または培養・加工を施したものを中間体(細胞ストック)として長期保存(凍結保存等)することが多くの場合可能であるため、自家細胞と比較して治療にかかる時間とコスト、そして品質のばらつきを抑えることが可能となり、実用化に適しているといわれています。また、実用化をさらに加速させるために、拒絶反応の原因となるHLAの一部を遺伝子編集により破壊したiPS細胞の準備が進んでいます。
網膜色素変性(RP)は、遺伝的背景により暗所での視機能を司る桿体視細胞が進行性に変性する疾患群であり、さらに進行すると中心視野、視力、色覚など司る錐体視細胞も2次的な変性をきたし、完全失明に至ることもある疾患です。現在では250もの原因遺伝子が報告されていますが、それらの遺伝子機能が解っていないものが多いです。その遺伝形式については常染色体優性、常染色体劣性、X連鎖性など、原因遺伝子により全ての形式が報告されていますが、家系内に他の発症者が確認できない孤発例もあります。
日本国内で網羅的な遺伝子診断スクリーニングにより診断がつくのは4割から5割とされています。RPは国の指定難病の一つであり、その罹患者数は4000人から8000人に1人とされ、日本国内では約3万人、世界的には約300万人と言われています。この病気では、多くの場合、最初に現れる症状は、暗いところでものが見えにくくなる夜盲です。その後、見える範囲(視野)が周辺部分から中心に向かい狭くなっていく視野狭窄が少しずつ進行していきます。さらに症状が進むと、視力の低下や、色の区別がつきにくくなる色覚異常が起きてくることが多いです。病気が進む速さは患者さんによって異なります。また、症状の組み合わせや順番にも個人差があり、最初に視力の低下や色覚異常で発見され、後から夜盲を自覚する患者さんもいます。この病気では、長い経過の後に視力がかなり下がる方は多いですが、完全に見えなくなる方はそれほど多くありません。
RPには現在確立した治療法はなく、症状を和らげたり、進行を遅らせたりする効果を期待して、遮光眼鏡(サングラス)の使用や、ビタミンAや循環改善薬の服用などが行われることがあります。また、将来的な治療法として、遺伝子治療や人工網膜などの研究が進められています。しかしこれらはまだ研究段階であり、今のところ、広く誰に対しても行うことができる治療法とはなっていません。そこで私たちは、iPS細胞から作製した視細胞を含む網膜シートを、視細胞が変性した患者さんの網膜に移植することにより、視覚機能を回復させることを目指す新しい治療法を、開発しています。
Cells utilized in regenerative medicine, such as somatic stem cells, embryonic stem cells (ES cells), and induced pluripotent stem cells (iPS cells), are characterized by their self-replicating capabilities and pluripotency. ES cells are stem cells established from a cluster of cells within a blastocyst following fertilization, and they can differentiate into almost all types of cells (tissues). In 2006, iPS cells were induced from mouse fibroblasts using four transcription factors (Oct3/4, Flk1, Sox2, c-Myc) by Professor Shinya Yamanaka of Kyoto University, serving as ES cell-like pluripotent stem cells. The following year, the same group succeeded in creating human iPS cells, which are now widely used not only in medical fields such as regenerative medicine and drug development support, but also in basic research such as rejuvenation, aging processes, and elucidation of cell differentiation mechanisms. On the other hand, adult somatic stem cells exist in living tissues, are maintained in a special niche microenvironment, and are believed to be involved in tissue regeneration by replacing cells lost during development and tissue damage.
ES細胞、iPS細胞、体性幹細胞などの幹細胞は、自己複製能と多能性を特徴としています。ES細胞は、受精後に胚盤胞内の細胞塊から分離して確立された幹細胞であり、ほとんどすべての細胞(組織)に分化することができます。 2006年、ES細胞様多能性幹細胞として作成されたiPS細胞は、京都大学の山中伸弥教授により、4つの転写因子(Oct3 / 4、Flk1、Sox2、c-Myc)を用いてマウス線維芽細胞から誘導されました。翌年、同グループがヒトiPS細胞の作製に成功し、現在、再生医療や創薬支援などの医療分野だけでなく、若返り、および老化プロセス、細胞分化のメカニズム解明などの基礎研究にも幅広く活用されています。一方で、成体体性幹細胞は生体組織に存在し、特別なニッチの微小環境で維持され、発達過程および組織損傷のために失われた細胞を補充することによって組織再生に関与していると考えられています。
RPE plays various vital roles in maintaining our vision, and it’s known that genetic abnormalities expressed in RPE, along with pathological changes due to aging, such as oxidative stress and abnormal accumulation of waste, can lead to RPE dysfunction. This dysfunction can cause a group of inherited diseases known as retinal pigment degeneration and Age-Related Macular Degeneration (AMD), which has been the focus of our previous clinical trials on RPE cell transplantation. In fact, several retinal degenerative diseases exist for which RPE dysfunction is the primary cause. Consequently, these retinal degenerative diseases are collectively referred to as RPE dysfunction (1). This umbrella term includes diseases such as crystalline retinopathy, an allied disease of retinal pigment degeneration, and those retinal pigment degeneration disorders accompanied by RPE-related genetic abnormalities (abnormalities in genes such as RPE65, RDH5, MERTK). These rare inherited retinal degenerative diseases, some of which are listed as specific intractable diseases, along with AMD, a common cause of blindness in the elderly, are all encompassed under RPE dysfunction. AMD affects about 700,000 people in Japan and approximately 300 million worldwide. However, the number of patients with other diseases categorized under RPE dysfunction is not known. Symptoms of RPE dysfunction are identified when visual acuity decreases, vision becomes impaired in dim light, or color perception becomes difficult. The age of onset and the type and progression speed of symptoms vary with the disease type and individual differences among patients. Age-Related Macular Degeneration is a typical example of RPE dysfunction.
Reference: